1 - Stratigraphy and Me. I imagine stratigraphy as a large book to leaf through, with thousands of pages and millions of words, symbols, and traces of previous readings and of previous readers. Pages that can be read by people who speak different languages, who are interested in different concepts expressed by the author, and who have very different skills of interpretation and different levels of education, and who are interested in different topics that are dealt with in the book. It is a book for readers who have experience in a variety of disciplines and who use the most advanced reading and comprehension techniques possible.
This book is to be read from the bottom up – from the last page to the first – because the layers of the subsoil accumulate one on top of the other and each one tells us about the process that created it, about the time when it was deposited, and about the climate, the environment, the plants, and the communities that wrote it. The gradual sedimentation of a new layer conceals and covers the information contained in the previous one, even though much of it remains within the same layer. We can imagine these layers as the pages of the book and the information contained in each layer as the written words, with the folds of the pages, the smudges of ink, and the erasures. As we go down into the subsoil, we can see that the pages are written in increasingly ancient periods and that if we compare “books” from different places, we will see that many more pages were written in some particular areas over a given period: this is where the geological processes were far more intense and thus left more traces. Our ability to read all the pages and to work out the real meaning of all the words depends on many factors.
One of the methods used to “read” the layers is that of comparing the sediments created by today’s natural processes with similar ones that we can see between the layers of the subsoil. If we look at them very carefully, we can see that they contain a lot of information that might be different from what we find today. For example, in the sediments deposited on the seabed we find that the sea was populated by species other than those we find now, that the environment was warmer or colder, or that the remains of plants or pollens in the sediments came from species that were different from the ones we find in the area today. Or that where a city now stands was once a swamp and, before that, that the area was covered by the sea.
Yet another problem is that of establishing the period when the various layers were formed. Stratigraphy enables us to establish which ones were deposited before and which ones came after, and this gives us their relative age. If we want to establish their absolute age, we can make a number of measurements: one of these is the analysis on the fossil remains of animals that lived only in a certain period, such as dinosaurs, and that left their traces among the rocks. Another is that of analysing objects whose age we know (such as ancient coins or vases and other objects that were produced and used by particular communities) and that were then buried by the deposits of volcanic eruptions. In these cases, the age of the subsoils can be considered as contemporary with, or later than, that of the objects themselves. Since the 1950s, other methods have been developed that make use of the presence of some radioactive elements in the rocks: these have a very precise decay history that can be measured in the laboratory. This gives the absolute age of the rocks, which is becoming increasingly accurate as we acquire greater knowledge and more advanced technologies for such measurements.
Let us now assume that we have studied the exact stratigraphy of the subsoil in a given area and that we have listed the events recorded in the strata. If we wish to compare the geological history of this place with others that may be close or far, we need to correlate the geological events of the individual areas. We will find that the same events were recorded at the same time in different settings: sedimentation in the sea, in lakes, or in alluvial plains, or areas of erosion, where the processes do not cause sediments, but remove the materials, thus possibly erasing the traces of previous events. These events can be synchronised by dating the strata or by correlating the sequences of rocks by using some elements they have in common. We can thus correlate similar and coeval levels, but in many cases we will see that, even in the same place, the strata may have slightly different characteristics, caused by small variations in the rock itself or in the environment where it was deposited. One example is that of lava flows that run down valleys, changing their characteristics very rapidly depending on their speed, on the presence of obstacles, of surface cooling and many other factors, even though they are all generated by the same magma. We could say that a lava flow is a single, consistent event, and one that is very rapid in geological terms, but if we look closely at how it moves – and the many videos of the flows on Vesuvius, Etna, or in Hawaii come to mind – we can see that each portion of the flow has its own story to tell: it may have hit a tree, covered a road, destroyed a house, or set fire to a wood, and just next to it, in the places it did not reach, there will be no trace at all of lava. Another example can be seen in volcanic eruptions – and particularly in large explosive eruptions such as the Plinian type. These eruptions create ash deposits that are spread over thousands of square kilometres. Their traces enable us to date the sequences of rocks in areas that are extremely far apart in a very precise manner. These deposits, which very quickly become sedimented in lakes, in the sea, in the mountains, in ice, in caves, and so on, allow to correlate – in other words, to regulate the sedimentation clock – over vast areas.
Let’s imagine we are observing strata from different parts of the world. We will discover that there are areas where some deposits cover huge areas, such as those of ash caused by large explosive eruptions – some may be very deep, while others will be very close to the surface, and in yet others they might not appear at all. This is because the geological processes that lead to the formation of the strata and their size can vary considerably in different depositional environments. For example, in an alluvial plain there will be thick deposits produced by flood waters that come one after another, covering the ash deposit we are studying, while on the surrounding hills this ash, if it is preserved at all, would be very close to the surface. But then, if we carry out our surveys in the sediments of a nearby lake, we will find that the layer of ash has been very well preserved and covered by the thin sediments of the lake. This means that depth alone is not enough to correlate events that took place at the same time in the past, so we need to synchronise our “clocks” correctly.
2 - Is stratigraphy an exact science? No! This negative answer is confirmed by my own experience, as well as by the advances in geological and volcanological studies that have been made in recent decades. Our ability to reconstruct geological history as indicated by the stratigraphy depends on:
- personal expertise,
- the complexity of the geological situation,
- advances in knowledge,
- the availability of new technologies,
- an understanding of past and present processes that form some deposits and similarities between modern and ancient strata, in their relative settings.
This means that our model – the history we read into the strata – will be the best summary possible today, making use of the knowledge and technologies we have at our disposal right now. If we analyse a rock, or a sequence of rocks and find a hypothetical process that has never been seen before by man, we may be led to adopt different interpretations about the stratum. We can, of course, apply new technologies or introduce new analyses that were not available before in order to increase our ability to interpret the strata correctly. Especially in the recent past, our interpretations have benefited from a multidisciplinary approach to sector studies. Different experts can read the same book and together grasp all the nuances of its content – just as different people looking at the same painting might be attracted by different aspects and be able to identify different elements: the painting technique, the style, the subject depicted, the context, the skill of the artist, and the materials used. All of this in a single image, which is the result of a complex, often unrepeatable process.
3 - An imaginary journey into the stratigraphy of a volcano. Also the history of a volcano is a book to leaf through. It contains traces of great eruptions, long periods of quiescence, low-energy eruptions, the interaction of the volcano with its surrounding area and, often, with the communities that have chosen the area around the volcano as a place to live and cultivate, and to build their villages and their cities, even though this may seem quite incomprehensible. But the reason is actually easy to understand, for most of the life of a volcano (such as Vesuvius) is one of long periods of inactivity that are often longer than a human lifespan. The great benefits of living in a volcanic area near the sea, however, have always been of great appeal to communities.
The journey...
I leave Naples behind me and go up Mount Vesuvius. On my way, I come across many types of volcanic rocks: lava flows, banks of ash, layers of light-coloured pumice and dark scoria, and various types of tuff. And yet I can never work out when they were produced by the volcano and in what sequence. I would like to know the history of the volcano and imagine its eruptions. As I go up the slope, I find the road is becoming increasingly steep: I started out on the flat and now I’m climbing up a volcanic cone. I go all the way to the huge chasm of the crater. I look inside and recognise some of the rocks I saw on the way up. I see a sequence of banks of dark lava and layers of reddish scoria. In various places the volcano is emitting hot, acrid-smelling steam. I measure its temperature and find it is over 100 degrees Centigrade. I look at my geological map and I realise that the rocks I have seen are very young, just a few hundred years old. They were produced by eruptions of low explosive power, like those of Etna: lava flows, lava fountains, and Strombolian explosions. The last one was in March 1944. The explosions that came in the most intense phases of the eruption formed the large crater I see before me, which is more than 300 metres deep and 700 wide (Fig. 1). I’m intrigued by a little building on the opposite side of the crater rim, and from a distance I can see it has a sign, but I can’t read it. I go up closer and then I read: ascensore stratigrafico – macchina del tempo(“stratigraphic lift – time machine”). That’s curious – I didn’t know about this new invention. I cross the threshold of the little building and find I’m on the top floor. The indicator light says 1944: we are on the layers produced by the eruption. The doors open and I see a push-button panel that has dates in place of floor numbers. I recognise them – they are the dates of the eruptions of Vesuvius.
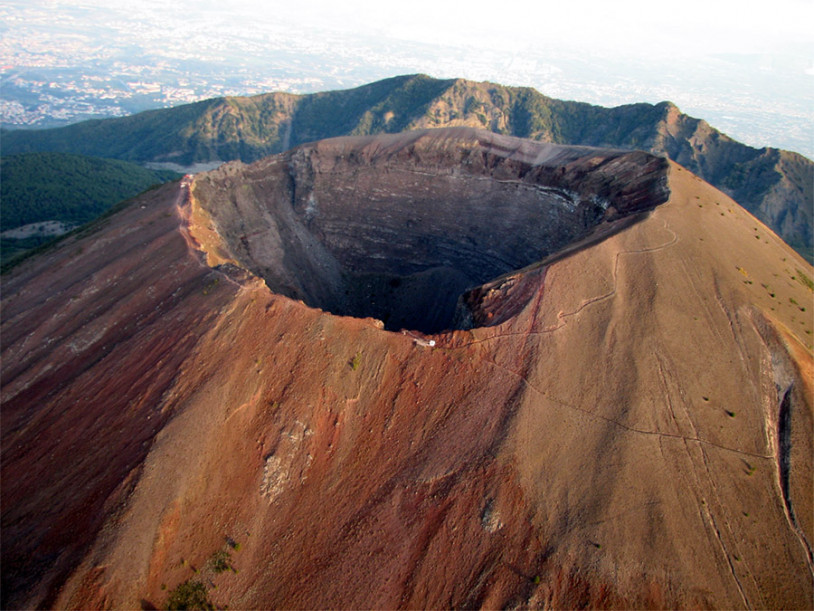
Fig. 1. Crater of Vesuvius, with the rim of the caldera of Monte Somma behind.
In the lift cabin I see buttons with the dates 1906, 1631, 472, 79, 1900 BC…, with the bottom floor at 40,000 years ago. That must be the date when the volcanic cone of Monte Somma, the oldest part of the volcano, started to rise up.
I press the 22,000 button and enter a succession of lava and scoria flows. It seems to me that the volcano is much higher than it is now and there is considerable activity all around me. Something huge and catastrophic is happening: a Plinian eruption, but this is the first – the oldest. I can see an eruptive column rising to a height of at least 30 kilometres from the volcano, scattering ash and pumice over a really vast area. The eruption goes on for a few days and the quantity of material ejected is in the order of cubic kilometres – so much so that the magma chamber, which can’t be reached by the lift because its temperature has reached almost a thousand degrees, is almost completely emptied. A piece of the volcano – the highest part of the cone – collapses on itself and forms a caldera (Fig. 2). There are powerful seismic shocks, the ground never stops shaking, the air is unbreathable, there’s ash everywhere and it’s burning hot, but I’m curious, so I resist. I want to get to the end. I see that the highest part of the volcano has collapsed and disappeared. The volcano now has a large elliptical depression at its centre, with a steep escarpment all around. I recognise it – I was looking at that escarpment from the edge of the crater, before entering the lift (Fig. 3).
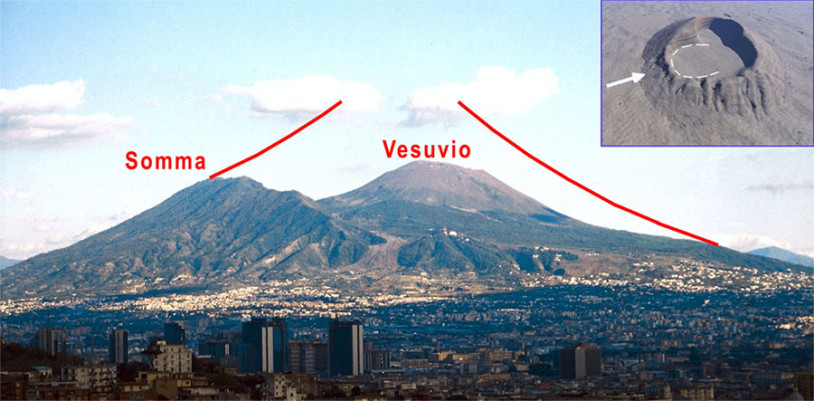
Fig. 2. A tentative reconstruction (in red) of the shape of the Somma before the caldera collapse 22,000 years ago. In the box, a bird’s eye view.
When I recover from the fright, I look at the push-button panel again and see that many of the buttons are lit up in green. They seem reassuring. I press one that doesn’t contain a date but a whole period, 6000-1950 BC. The lift takes me to the slopes of the volcano, and I look out over its lush green slopes. It’s not active now. There are cultivated fields all around me, with lots of animals, hunters, and farmers with ploughs being pulled by animals, while others are taking their flocks out to graze. Everything has changed since the devastation I witnessed earlier on: the volcano is giving back life, fertilising the soil, attracting communities. I take a closer look and discover that there are also streets and clusters of huts made of wood and straw (Fig. 4). I consult my geological map and leaf through the pages of the book, only to discover that all this won’t last much longer. The volcano is preparing for another Plinian eruption – the one that will take place at the end of the Early Bronze Age and that will devastate the entire area around the volcano for thousands of square kilometres, sealing everything I’ve seen underground for ever. Archaeologists and volcanologists will find traces of these ancient civilisations only 4,000 years later.
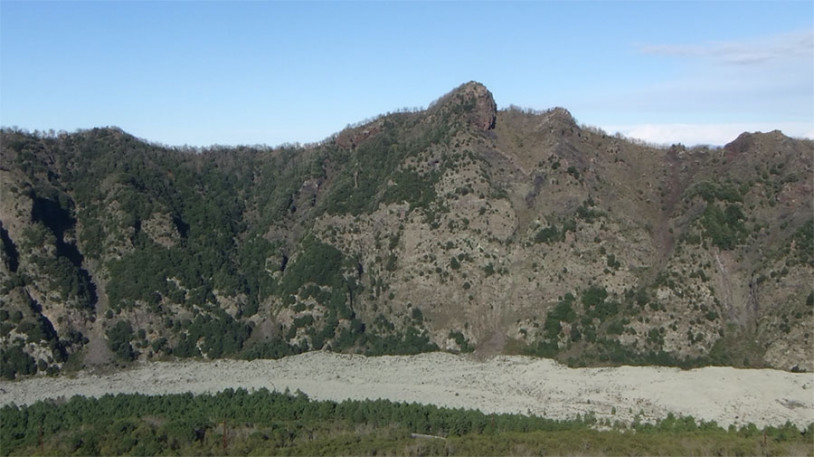
Fig. 3. The escarpment of the caldera on Monte Somma. The light grey lava flow of the 1944 eruption can be seen at the foot of the escarpment. The rocks produced during the ancient activity of Monte Somma, consisting of lava flows and scoria from low-energy explosive activity, can be seen in the escarpment, which formed as a result of the calderic collapse. At the centre is Punta Nasone, which is also visible in Fig. 1.
The eruption in the Early Bronze Age intrigues me. I’ve studied it, and now I want to see it live. I press the 1950 BC button with decision. The eruption doesn’t look that different from the one of 22,000 years ago: a high eruption column, only this time the ash and pumice fall towards the northeast, towards the area where Avellino will be built. Thick blankets of ash and lapilli are piling up on the ground. Many villages have already been destroyed, and the landscape is unrecognisable, the sky invisible. The eruption has already been going on for several hours: huge blasts, earthquakes, unbreathable air. But then suddenly it all becomes even more intense. I look towards the northeast, where things appear to be calmer, and I find there are whole communities fleeing, with their herds, small supplies of food and some valuables, many of them in bronze. They are moving northwest, going away from the volcano. They’re walking on the hot ash that’s just fallen, leaving their footprints behind. They move in groups, with adults, children, and animals. Many are dragging objects. Looking closer, I see they’re going past a village of huts that’s partially destroyed. Someone goes into a hut. Today, that village is just next to what is now the high-speed railway line and the holes for the hut poles, and traces of their escape and of the devastation can all now be seen, preserved in the stratigraphy of the eruption deposit (Fig. 5). The entire area around the volcano will remain almost uninhabited for a few hundred years.
Tired, but increasingly curious, I press the AD 472 button. The Roman Empire is almost coming to an end. The area is in decline and many of the sumptuous villas around the volcano are in a state of partial disrepair. There are a lot of seismic shocks, and there is no water in the Roman aqueduct leading down from the nearby Apennines. The walls of the houses, some of which have been abandoned, are beginning to collapse. The volcano is swelling, with magma welling up from the depths, and eruption is imminent. Massive explosions begin, and a mixture of fragments of magma and gas is ejected from the volcano conduit at supersonic speed, rising tens of kilometres into the atmosphere. Giant volcanic blocks and bombs are hurled from the mouth of the volcano, raining down like projectiles, smashing through roofs and penetrating the ground. The cloud fed by the column heads northeast, like that of the eruption thousands of years earlier, and it covers a vast area, with its centre over Nola. The amphitheatre, the basilicas, and the entire city are covered with a thick layer of lapilli. Suddenly the explosions no longer support the eruption column and it collapses on itself, generating scorching pyroclastic flows that run down the sides of the volcano all the way to the surrounding plain, submerging everything they encounter. Their temperature is extremely high – over 300 °C. A huge amount of ash and pumice ends up on the volcano, on the plain, and in the Apennines. The volcano has pushed out an enormous amount of steam, which condenses, creating heavy rainfall. The ash that has piled up turns into mud and begins to flow, flooding the plain. This muddy mass fills everything – valleys, streets, houses and monuments. The phenomena go on for many months, making a vast area around the volcano uninhabitable.
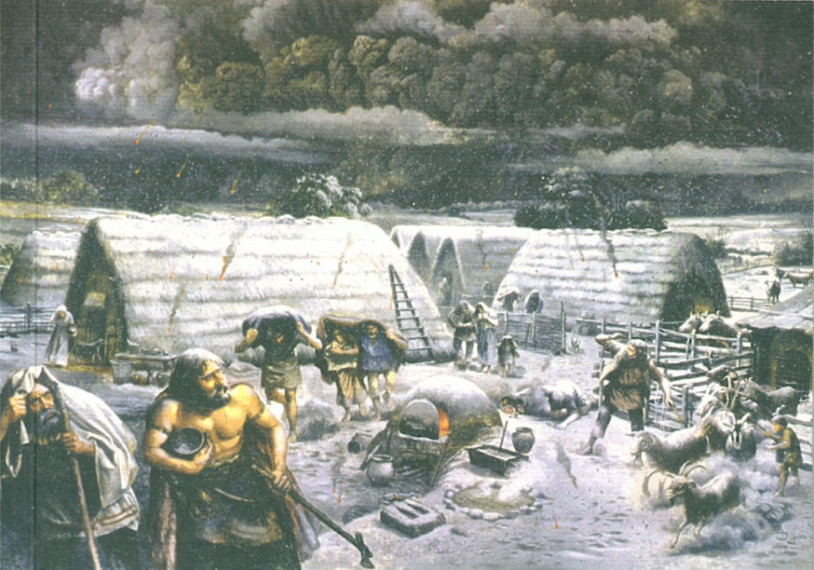
Fig. 4. Reconstruction of life in an Early Bronze Age village at the moment of the Early Bronze Age eruption of Vesuvius (modified from the cover of Il villaggio del Bronzo Antico di Nola, Litografia Sicignano, Pompeii, 2005).
I’m exhausted, so I press the 0 button, and come back out from the depths. I’ve come back to the present year. I meet my fellow volcanologists, who are surveying the volcano. They are installing stations to measure the gas that feeds the fumaroles, to record earthquakes, and to see if the volcano is changing shape, so that they can monitor its state. I look out at the volcano again. The traces of the last eruption, the one that came in 1944, are fading away. Vegetation is covering over the slopes of the volcano. I’ve already seen a landscape like this on my trip. The volcano is not extinct, but just sleeping right now. I’d love to have a lift that could take me up into the future, all the way to the next eruption, but I know that, at least for the moment, that part of the lift hasn’t yet been built.
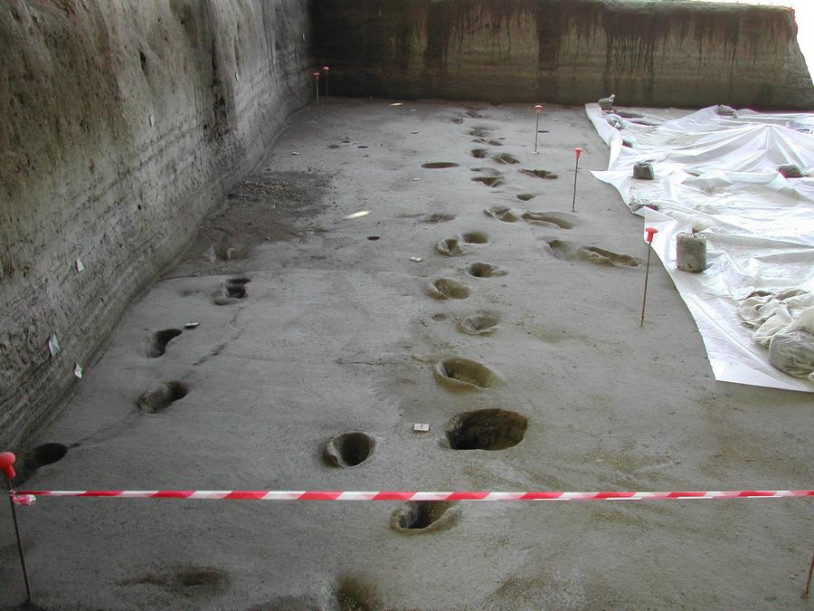
Fig. 5. Footprints of people fleeing, left in the ash during the eruption of Vesuvius in 1950 BC.